Sailing to the Stars on a Beam of Light
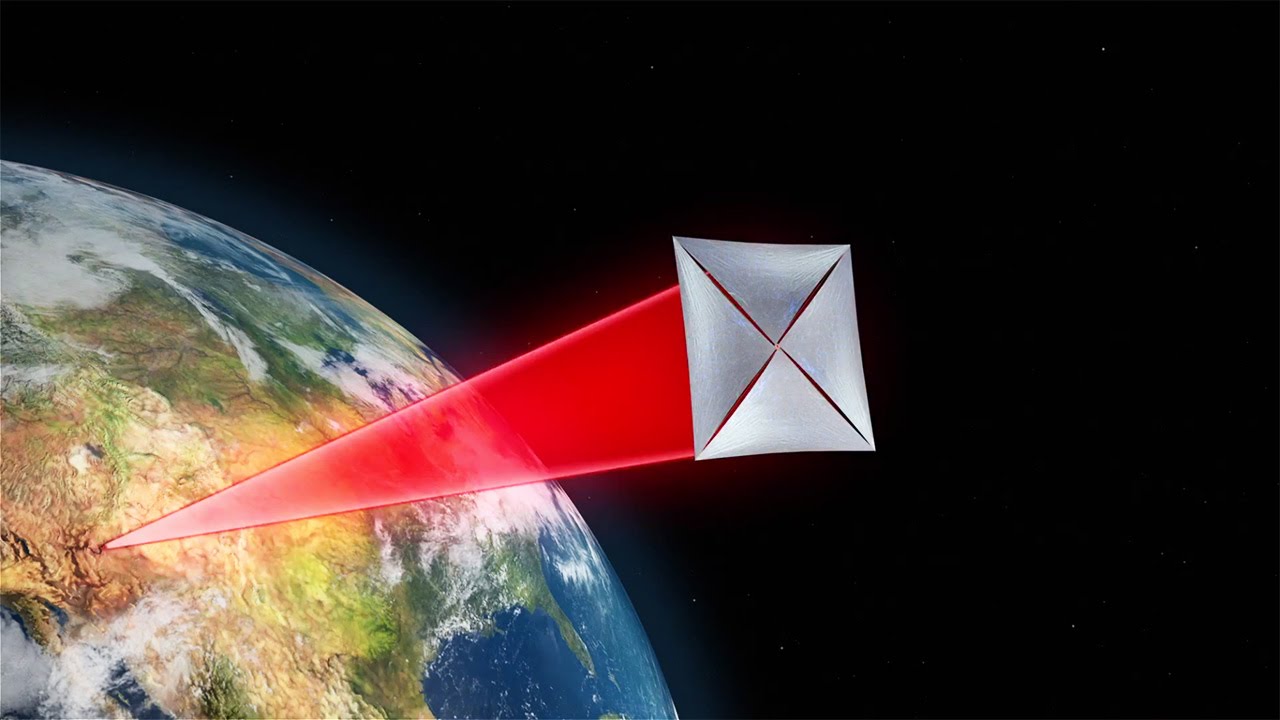
How existing technology can send a spacecraft to the nearest star in less than a human lifetime.
Mark Baumann
November 30, 2022
This is part four of a four-part series on interstellar spacecraft. My goal at the outset was to address the question: is it possible to build a spacecraft that could travel from here to our nearest neighboring star, Proxima Centauri, in less than a human lifetime?
In the previous three articles we’ve considered fusion-powered spacecraft and spacecraft propelled by nuclear explosions, as well as more exotic concepts like Bussard Ramjets and matter-antimatter propulsion. Every option considered so far has either been too slow to get us there in a human lifetime, or impossible to build with current or near-future technology, or both.
In the previous article I introduced the “spacecraft fuel problem”: To accelerate to speeds that allow travel at interstellar distances, most of the spacecraft needs to be fuel. Even a rocket which just gets us to Earth’s orbit is 85% fuel, for example.
This article presents a possible solution to this conundrum, which is to build a spacecraft that carries no fuel at all. We can leave the fuel and the propulsion system itself at home and send the energy from home to the spacecraft. This could be achieved using a beam of electromagnetic radiation, or light.
Light is Strange
Light is really strange stuff. For example: it’s the fastest stuff in the Universe; nothing can go faster. Light has no mass. Every observer measures the speed of light to be the same, no matter what their frame of reference. Light can act like a particle, but it can also interfere with itself like a wave. The list goes on.
Light is so interesting that Einstein reached many of his insights by studying and thinking about light.
Light has another curious feature that is relevant to the topic of interstellar propulsion: Light has no mass, and yet it carries momentum.
If an astronaut were floating in empty space and possessed only a flashlight, the flashlight could be used as a means of propulsion. Turning on the flashlight, it ejects photons (particles of light) in the direction it’s pointing. Each photon carries away some momentum, which in turns gives a momentum boost to the astronaut in the opposite direction. As if wielding a machine gun that fires incredibly small pellets, the astronaut would experience the “recoil” from the flashlight and be propelled backwards by it. Using just a flashlight, the propulsion felt by the astronaut would be incredibly small but it’s there nonetheless.
Another way to achieve the same effect would be to have the flashlight (affixed to some other object) shine on the astronaut. This way, rather than ejecting photons, the astronaut is catching them. The momentum carried by each photon is absorbed by the astronaut when the photon is absorbed. This provides a push on the astronaut with the same force as the recoil the astronaut would experience when holding the flashlight in hand.
This scenario of the astronaut being pushed by photons is analogous to a sailboat with wind in its sails. The wind pushes on the sailboat’s sails, transferring momentum to the sailboat and thus providing propulsion. In the case of the astronaut, light is acting as the “wind”.
Even better than absorbing photons, the astronaut can get an extra boost by reflecting them (using a shiny object like a mirror, for example). To understand why reflecting is better than absorbing, let’s use another analogy.
Imagine you are standing on a perfectly slick ice skating rink and you throw a ball. The act of throwing the ball will cause a recoil that will cause you to slide backwards on the ice. This is analogous to the flashlight ejecting photons in one direction to provide thrust. Now imagine you catch a ball that’s thrown to you and then throw it back. You would get two momentum boosts backwards: one boost from catching the ball and absorbing its momentum, and another boost from the recoil of throwing it back. This is analogous to a mirror that reflects photons: the mirror must first “catch” the photon and then “throw” it back. Therefore, reflecting the photon gives twice the momentum boost that absorbing the photon does.
Another way to boost the effect of the photon thrust is to use a more powerful light source. A more powerful light source emits more photons (produces more “wind”). So a searchlight would be more effective at providing a push than a handheld flashlight. What about a really powerful light source, like the Sun? Or a laser? Let’s investigate both of those possibilities.
Sailing the Heavenly Breezes
“Provide ships or sails adapted to the heavenly breezes, and there will be some who will brave even that void.”
-
- –Johannes Kepler (1610)
The idea of a solar sail has been around for 400 years, at least since Kepler (best known for his three laws of planetary orbits) wrote to his friend Galileo in 1610 with the idea to sail on “heavenly breezes” (quoted above). His idea was inspired by the observation that all comets have a tail that points directly away from the Sun, no matter in which direction the comet is moving. This led him to theorize the Sun produces a “wind”.
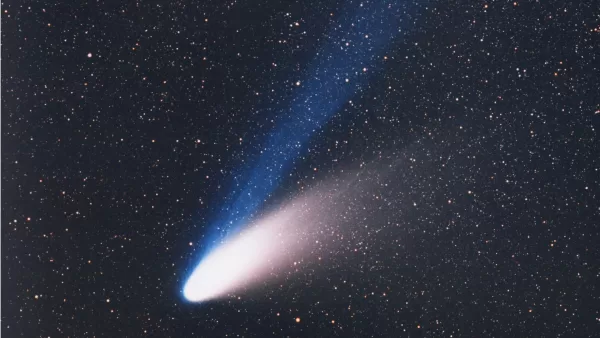
The two tails of a comet. (Image credit: ESO/E. Slawik)
Comets have two tails (as seen in the image). One is made of dust particles that break off from the comet and leave a trail in the comet’s path (the white tail in the image). The other is a narrow tail of ions that always points away from the Sun (the blue tail), as noticed by Kepler (and Tycho Brahe before him).
While it’s true that there is a solar wind, which is a stream of charged particles that have been ejected from the Sun’s corona, it’s not the solar wind that directs a comet’s ion trail away from the Sun. Instead, that’s caused by the Sun’s magnetic field, which is so expansive that it encompasses the entire solar system. However, Kepler’s idea of sailing on the energy provided by the Sun is still relevant.
At Earth’s distance from the Sun, absorbing the momentum of the solar wind’s particles (which amounts to a pressure of 1-6 nanopascals) is about 1000 times weaker than absorbing the momentum of the Sun’s light (amounting to about 4.5 micropascals). Therefore, sailing on the Sun’s light rays would be much more effective than sailing on the solar wind.
A sail pushed by the force of the Sun’s light is the concept behind a solar sail.
Constructing a Solar Sail
Solar sails are built of a lightweight, reflective material. The first solar sails have already been built and deployed.
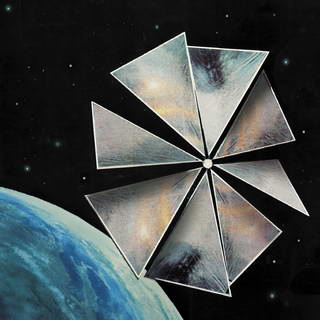
Cosmos 1 solar sail (artist’s rendering)
The first attempt at deploying a solar sail was the Cosmos 1 mission in 2005 (artist’s rendering pictured). The sail consisted of eight sail blades, each 15 meters long, built of Mylar (a lightweight, reflective material). The spacecraft launched on June 21, 2005 onboard a Russian rocket. Unfortunately, due to a rocket malfunction, the sail never reached orbit.
The first successful solar sail was IKAROS in 2010, a project of the Japan Aerospace Exploration Agency. IKAROS has a square sail measuring 20 meters on the diagonal (a total of 200 square meters) and made of a layer of polymer that is only 7.5 micrometers thick — less than the thickness of a human hair. After launch it proceeded on its six-month voyage to Venus and successfully passed Venus on December 8, 2010.
Following up on their failed Cosmos 1 mission, the Planetary Society developed another solar sail spacecraft called LightSail. LightSail was crowdfunded on Kickstarter. Like IKAROS, LightSail uses a square sail. It measures about 32 square meters in area (significantly smaller than IKAROS), or roughly the size of a boxing ring. The sail has a thickness of 4.5 micrometers (even thinner than IKAROS).

View of Earth from LightSail 2
LightSail has consisted of two missions. LightSail 1, launched in 2015, was a mission designed to test the deployment of the sail and it never actually sailed on sunlight. LightSail 2, launched in 2019, was a fully-functioning solar sail that orbited the Earth for over three years.
LightSail 2 orbited at an altitude higher than that of the International Space Station. Even at that altitude, though, there is enough Earth atmosphere to produce drag, which causes the spacecraft to lose altitude. By turning its sails to face the Sun, it would receive a boost that raised its altitude. With its sails sometimes facing the Sun and sometimes not, it sailed in an undulating, sometimes-lower-sometimes-higher orbit around the Earth.
You can view LightSail’s status updates and see a plot of its changing altitude with this online dashboard. As this article was being written, LightSail 2 re-entered Earth’s atmosphere on November 17, 2022 and burned up, successfully completing its mission.
NASA has three future solar sail missions planned: NEA Scout, Solar Cruiser, and ACS3.
The history of solar sailing is nicely summarized in this infographic below from the Planetary Society.
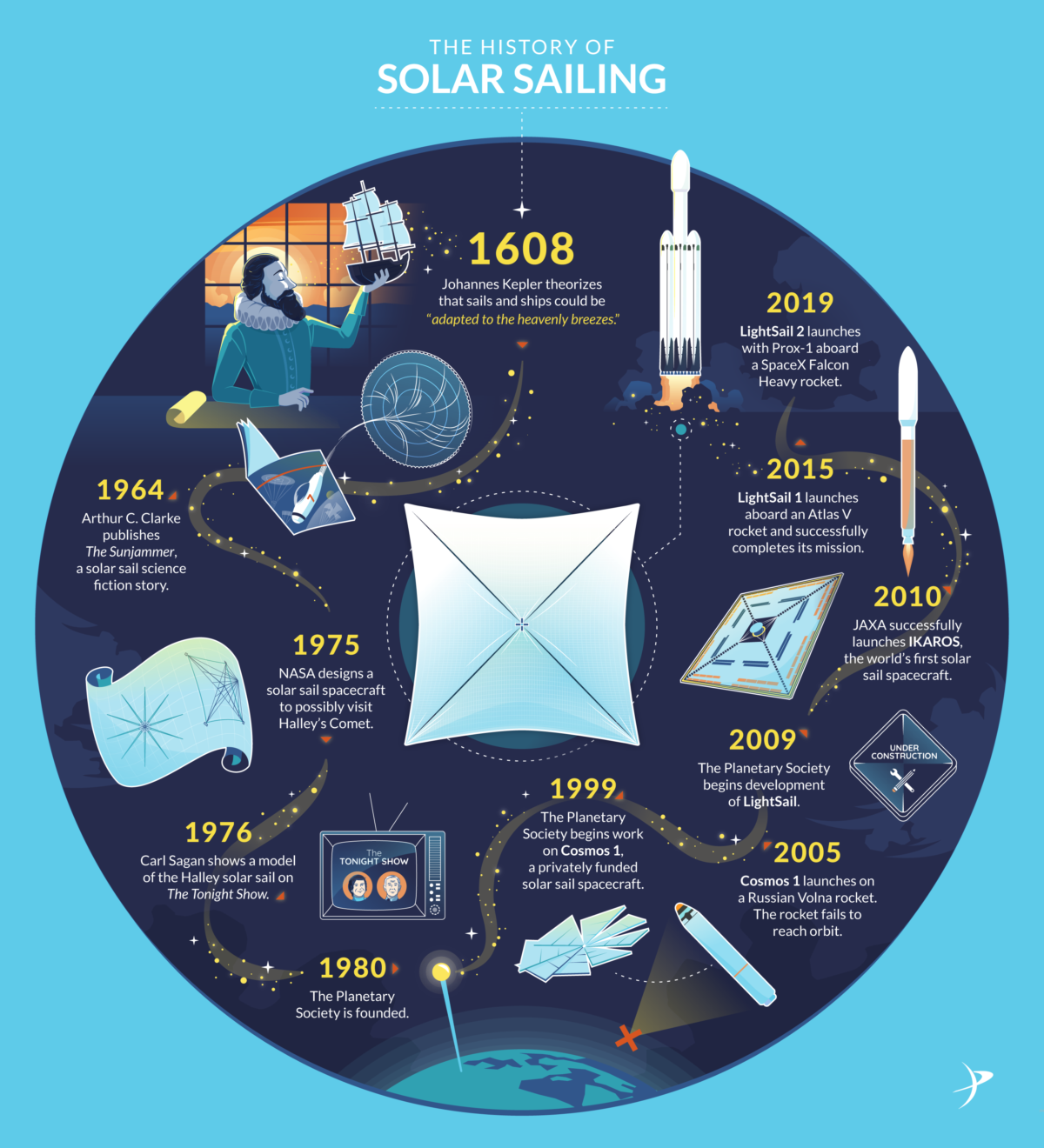
Image credit: The Planetary Society
Sun-Diver
Could a solar sail leave the solar system? A strong boost from the Sun would be required to achieve the needed speed. Getting a stronger boost from the Sun would involve building a bigger sail, getting much closer to the Sun, or both.
The Sun-Diver mission was proposed by Greg and Jim Benford in 2003. [1]Benford and Benford, “Near-Term Beamed Sail Propulsion Missions: Cosmos-1 and Sun-Diver”, AIP Conference Proceedings 664, 358 (2003) (The word Sundiver was used prior to 2003 as the title of David Brin’s 1980 sci-fi novel Sundiver. In an interview with Greg Benford in Fantasy and Science Fiction magazine, Benford said he gave the name to Brin “when he first came to see me, back when he was struggling with his first novel.”[2]https://www.centauri-dreams.org/2017/06/05/parker-solar-probe-implications-for-sundiver/.)
The idea is to take a solar sail and send it edge-on towards the Sun, “diving” to a distance of just 20% of the distance from the Sun to the Earth. Once there, it turns face-on to the Sun and receives a boost from sunlight that is 20 times more intense than the sunlight we experience at Earth’s distance from the Sun. This boost from the Sun’s light would propel the sail away from the Sun with enough velocity to climb out of the Sun’s gravitational pull and escape the solar system.
Such a mission could reach Pluto in about 5 years and ultimately escape the solar system with an exit velocity of about 20 km/s. [3]Benford and Benford, “Near-Term Beamed Sail Propulsion Missions: Cosmos-1 and Sun-Diver”, AIP Conference Proceedings 664, 358 (2003) At that speed it would still take it nearly 64,000 years to reach Proxima Centauri.
A Sun-Diver mission would be an effective way of exploring the solar system, but it won’t get us to our nearest neighboring solar system in anywhere near a human lifetime.
For interstellar travel, we’ll need to consider an alternative with more focused light energy, like a laser.
Laser Sails
A laser sail is basically a solar sail that gets pushed by laser light instead of the Sun’s light. The concept of a laser sail was first proposed by Robert Forward in 1984. [4]R. L. Forward, “Roundtrip Interstellar Travel Using Laser-Pushed Lightsails”, J. Spacecraft and Rockets, 21, pp. 187-195, 1984.
No laser sail has yet been built but there are at least two proposed laser sail missions: Starlight, a NASA-funded project at the University of California, Santa Barbara; and Breakthrough Starshot, currently receiving only private funding from Yuri Milner’s Breakthrough Initiatives foundation. At the time the Breakthrough Starshot project was announced, the board of directors consisted of Yuri Milner, Stephen Hawking, and Mark Zuckerberg.
The name Starshot was inspired by the Moonshot nickname that was given to the Apollo Moon landing project.
Breakthrough Starshot
“Today we commit to the next great leap in the cosmos, because we are human and our nature is to fly.”
-
- –Stephen Hawking, at the announcement of Breakthrough Starshot
Breakthrough Starshot wants to send an extremely small probe over interstellar distances.
How small? About the size of a paperclip.
How can a spacecraft be so small? By solving the spacecraft fuel problem: it carries no fuel. Effectively, the spacecraft is just a computer chip. The chip would include cameras (CMOS imagers), communications (an optical laser), accelerometers, gyros, a photon thruster (an LED or laser), and a battery (powered by nuclear fission), all in miniaturized form. This technology is available to us now, and in some cases doesn’t differ so much from the technology in our smartphones. Take out the guts of a smartphone by removing the battery, screen, and user interface (the bulk of the phone), add a nuclear battery and some tiny lasers, and you have a spacecraft on a chip.
A spacecraft made out of a computer chip has been nicknamed a StarChip.
The idea is to attach the StarChip to a 5-meter-diameter laser sail, which uses technology similar to the solar sail technology we’ve already implemented. Then we propel it by shining a laser on the sail. The chip weighs only about a gram and the sail — being thinner than a human hair — weighs an additional gram. Since we’re leaving the propulsion system (the laser) at home, there’s no fuel to carry along. The resulting small mass of the chip plus sail means the spacecraft has very little inertia, making it easy to accelerate quickly to high speeds.
A 70 gigawatt laser shining on the laser sail for less than 10 minutes is enough to accelerate the sail to 20% the speed of light. During that 10 minute period, the spacecraft would experience 20,000 G’s of acceleration.
To give you an idea of how fast 20% the speed of light is: at that speed, the spacecraft could travel from Earth to Mars in about 30 minutes. To get to Pluto would take a little over a day. [5]It takes 5.5 hrs for light to cross the ~39 AU separating Earth and Pluto. The spacecraft is 5x slower than light, for a total travel time of 27.5 hrs
The spacecraft that has traversed the greatest distance from Earth is Voyager 1, followed closely by Voyager 2. Voyager 1 was launched in 1977 and, after traveling for 45 years, has reached a distance of 159 AU (that’s 159 times the distance from Earth to the Sun – or nearly 15 billion miles). Voyager 1 left our solar system on August 1, 2012 [6]https://solarsystem.nasa.gov/missions/voyager-1/in-depth/. (It was planned that the Voyagers would leave the solar system; they each carry the Golden Record, a message from humanity to any intelligent extraterrestrial that might find it.) You can monitor the position and status of both Voyager 1 and 2 using NASA’s dashboard.
At 20% the speed of light, a spacecraft from Earth could catch up to and pass Voyager 1 in four and a half days. [7]20% the speed of light is about 35 AU/day That is, the distance that took Voyager 45 years to traverse could be traversed by Starshot in 4.5 days.
To make the 4.4 light year journey to Proxima Centauri would take Starshot about 20 years, less than an average human lifespan.
Starshot promises to be the solution that we’ve been hunting for in this series of articles: it can cover the distance from here to Proxima Centauri in less than a human lifetime and do so using technology that exists or is within our grasp.
To see an animation of how all of this might look, here is a video that was released in 2016 when Breakthrough Starshot was announced:
Challenges
Of course, there are many technological challenges. I’ll discuss a few.
Laser Array
The numbers quoted so far have depended on a laser that can maintain a 10 minute output at 70 GW of power. No such laser exists today. However, that power can be achieved using an array of lasers, all working in perfect lock-step (this is depicted in the animation above). This technology has been successfully developed and tested in a lab.
The array is modular and can be any size. A bigger array, while more expensive and harder to build, leads to higher speeds.
As Philip Lubin, physicist and author of A Roadmap to Interstellar Flight, describes it, “This is radically different than the older ‘use a huge laser’ approach to photon propulsion. This is the equivalent to modern parallel processing versus an older single processor supercomputer.” [8]Philip Lubin, “A Roadmap to Interstellar Flight” [ pdf ]
Along the way to building a 70 GW array, one could build a smaller array that is capable of accelerating probes to speeds like 200 km/s (40 AU/yr), which would allow us to explore our more immediate neighborhood, such as the heliopause, Oort Cloud, Kuiper Belt, and more. Being modular, that same array can grow over time as elements are added, leading to ever more powerful arrays capable of higher speed flight.
The road to interstellar flight is not all or nothing; at every stepping stone along the way to interstellar flight there are many options for exploration with this technology.
Sail
Having a highly reflective sail is important for the efficiency of the momentum transfer from the laser to the sail. But it’s also important for the survival of the sail.
What keeps the sail from vaporizing under the intense radiation of the laser array? The answer: it must be so reflective that it absorbs almost nothing. If it doesn’t absorb any of the light, then the light can’t heat it up. State-of-the-art materials (like glasses designed for fiber optics) absorb as little as one part per trillion of incident light energy.
And what about all that light energy being reflected right back at the laser? It can be recycled by the laser array, improving the laser’s energy efficiency.
Marksmanship
To come within 1 AU (the distance from the Sun to Earth) of Proxima Centauri requires remarkable precision in the spacecraft’s flight path. While 1 AU may seem like a large target (1 AU is about 90 million miles), it is tiny relative to the nearly 270,000 AU that the spacecraft must cross to get there.
When initially accelerating the spacecraft with the laser, our aim will have to be remarkably accurate. Even a small error in the initial trajectory can amount to being off course by hundreds or even thousands of AU by the time the spacecraft has traveled for 20 years at 20% the speed of light.
Small course corrections could be made with a photon thruster. This is nothing more than a small laser onboard the StarChip that uses the momentum of light (like our hypothetical astronaut discussed earlier who uses a flashlight for propulsion) to adjust the spacecraft’s course. One-watt lasers that weigh less than a gram are widely available at low cost. [9]https://breakthroughinitiatives.org/forum/17?page=7
Interstellar Dust
At the blazing speed of 20% the speed of light, even a tiny dust grain could blow a hole in the spacecraft. A shield could be added to the StarChip, but it must be small to stay under the 1 gram limit. Another proposal is to build redundancy into the StarChip; with multiple copies of each onboard system, it could sustain several blow-throughs and still continue to operate at full capacity.
To help avoid dust particle collisions, the spacecraft orientation could be changed. This is another use for the photon thruster. The photon thruster can turn the StarChip on its side so that it will be edge-on as it flies towards its target, rendering its cross-sectional profile much smaller to help avoid collisions.
On the bright side, the momentum transfer of dust particle collisions to the spacecraft is a tiny fraction of the spacecraft’s total momentum, even when taking all of the expected collisions combined. So only minor course and orientation corrections are expected as a consequence of dust impacts, and these course corrections could be made by the photon thruster.
A Swarm of Probes
Another solution to the problems of aim and interstellar dust is to send not one spacecraft but many of them. That would greatly increase the odds that at least one intact spacecraft would come within 1 AU of Proxima Centauri.
Could we construct a swarm of spacecraft? The SpaceChip would be relatively cheap to produce. The most expensive part –- the laser -– is the part that we can re-use over and over again. The Starshot designers have suggested doing about a hundred launches per day, or roughly 40,000 per year. [10]Philip Lubin, “A Roadmap to Interstellar Flight” [ pdf ]
While we’re at it, we can send swarms of probes throughout our solar system. With the ability to reach Mars in 30 minutes and Pluto in a day, the solar system suddenly feels like a much smaller place to explore.
Deceleration
Carrying no propulsion of its own, the spacecraft will have no way to slow down.
Therefore, what’s being proposed are fly-by missions. Another use for the photon thruster arises: When the StarChip arrives at its target, the spacecraft can be continuously reoriented by the thruster to take photos as it zooms by.
Communications
Sending a message back over 25 trillion miles is no small feat. However, using short bursts of 1 watt transmissions, combined with a receiver with a very large area (such as the laser array itself), we could receive (in the best case scenario) as many as 650 data bits per second here on Earth even when the StarChip is at Proxima Centauri. At that data rate, it would take about 12 seconds to transfer a 1 MB image.
The bigger limiting factor in communication is the latency. Since Proxima Centauri is 4.4 light years away, it would take 4.4 light years for any message to cover the distance between us and the spacecraft.
Autonomy
On a related note, the latency of communication makes it impractical for this spacecraft to be remote-controlled. Any command we send it will be ~4 years old when it finally arrives at the craft. And then it takes another ~4 years for us to receive a response. There is no way around this speed of light limitation.
Therefore, the spacecraft will have to be autonomous. Using its onboard cameras and processor to monitor the positions of the stars, plus a photon thruster for course corrections, StarChip can steer its own course guided by the stars… just like a sailing ship of old, but this time piloted by an AI.
Battery
To keep the spacecraft at the scale of a gram, only 150 mg has been allotted for the battery. This tiny battery has to power every system onboard, including the 1W short bursts of communication sent back to Earth.
The proposal is to use a nuclear fission power source, either plutonium-238 or Americium-241. The decaying radioactive isotope will charge an ultra-capacitor. This is a much smaller-scale version of the plutonium-238 battery used by the New Horizons spacecraft that visited Pluto in 2015. [11]https://breakthroughinitiatives.org/forum/16?page=5
Human Spaceflight
So far we’ve discussed sending a gram-scale probe to Proxima Centauri using a laser sail. What about spacecraft larger than a gram?
The technology works the same for larger spacecraft. The bigger the laser array, the faster the final speed. But the bigger the spacecraft, the smaller the final speed. It’s a trade-off and we can choose where on the spectrum we wish to build.
For example, the 70 GW laser array discussed above could propel a 10,000 kg spacecraft (a medium-sized human-capable spacecraft) to more than 1000 km/s (0.33% the speed of light). Or a Space Shuttle class vehicle (100,000 kg) to a speed of 0.26% the speed of light or 780 km/s — at that speed astronauts could get to Jupiter in less than two weeks, to Saturn in about three weeks, and to Pluto in less than three months. That speed won’t transport humans to Proxima Centauri in a human lifetime, but it will enable humans to go anywhere we want in the solar system.
And 70 GW is not the upper limit. The system is modular and in principle it can be expanded to any size. A bigger array leads to higher speeds, and therefore there’s no limit on the final speed aside from the speed of light itself.
Conclusion
“A decade ago what we now propose would have been pure fantasy. It is no longer fantasy.”
-
- –Philip Lubin (2016)
We already possess the technology to send a spacecraft to Proxima Centauri in less than a human lifetime. We could do so using a gram-scale spacecraft attached to a laser sail that’s accelerated by a laser array.
Clearly, a gram-scale spacecraft won’t be sending humans to the stars. But it could send our “eyes and ears” to the stars, and that seems like the obvious place to start.
The challenges of human spaceflight are vast and haven’t been discussed here, and those challenges are probably best tackled with interplanetary travel first before we seriously consider sending humans on interstellar voyages.
In the meantime, we have the capability to reach Proxima Centauri in 20 years with a probe. In the words of Philip Lubin, project lead at UC Santa Barbara, “much remains to be done but it is time to begin.” [12]Philip Lubin, “A Roadmap to Interstellar Flight” [ pdf ]
This concludes my four-part series on interstellar spacecraft. You can read the other articles in the series by clicking here.
Don’t miss the next article! Please sign up for my mailing list.
Footnotes
↑1, ↑3 | Benford and Benford, “Near-Term Beamed Sail Propulsion Missions: Cosmos-1 and Sun-Diver”, AIP Conference Proceedings 664, 358 (2003) |
---|---|
↑2 | https://www.centauri-dreams.org/2017/06/05/parker-solar-probe-implications-for-sundiver/. |
↑4 | R. L. Forward, “Roundtrip Interstellar Travel Using Laser-Pushed Lightsails”, J. Spacecraft and Rockets, 21, pp. 187-195, 1984. |
↑5 | It takes 5.5 hrs for light to cross the ~39 AU separating Earth and Pluto. The spacecraft is 5x slower than light, for a total travel time of 27.5 hrs |
↑6 | https://solarsystem.nasa.gov/missions/voyager-1/in-depth/ |
↑7 | 20% the speed of light is about 35 AU/day |
↑8, ↑10, ↑12 | Philip Lubin, “A Roadmap to Interstellar Flight” [ pdf ] |
↑9 | https://breakthroughinitiatives.org/forum/17?page=7 |
↑11 | https://breakthroughinitiatives.org/forum/16?page=5 |