Interstellar Travel and the Incentive Trap
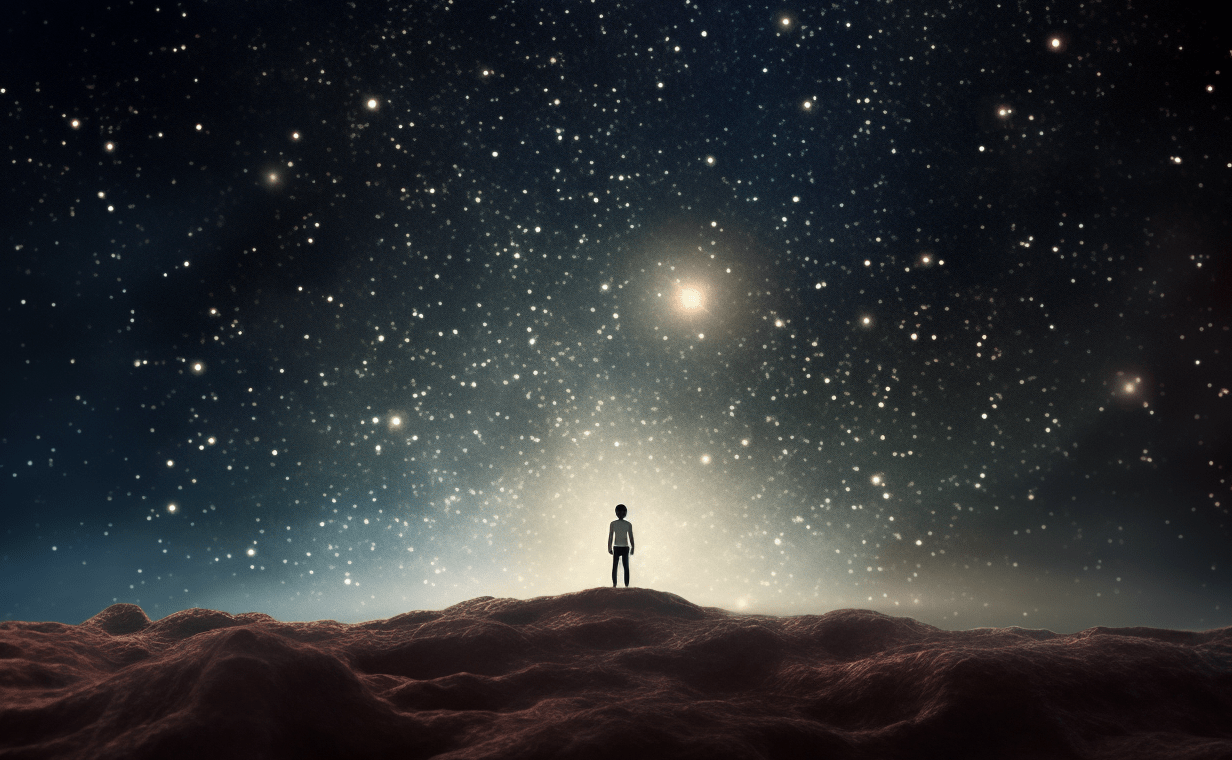
Should we begin our journey to the stars now, or wait until we have faster technology?
Mark Baumann
May 26, 2023
Travel to the stars has long captured the human imagination. Science fiction authors have been imagining trips to the Moon, planets, and stars since at least as early as 125 CE with Lucian of Samosata’s “A True Story”, a satirical story about riding a wooden ship to the Moon on a giant waterspout. Over time science fiction has become science fact and humans actually did visit the Moon (though not in a wooden ship!). We’ve also sent spacecraft beyond the Moon to other planets and their moons, and even out of our solar system. As of today, a total of five NASA probes have left the solar system. We are gradually getting closer to the dream of leaving this solar system and exploring another.
But, when will we be ready to travel to the nearest star? Should we go ahead and get started now, or wait until later?
The Incentive Trap
Suppose we could get to the nearest star in, say, 1000 years (that may sound slow, but a 1000-year travel time would require a starship that can travel at 0.4% the speed of light, or nearly 3 million miles an hour!). If we could do that now, should we build such a ship and send it on its way? (It would have to be a colony ship, as generations of people would live and die on the spacecraft during its 1000-year journey.)
The sooner we start, the sooner we’ll get there, right? Well no, not really.
We could wait until we have faster technology. Suppose in 100 years we will be able to build a spacecraft that can make the trip in 500 years instead of 1000 years. Then that spacecraft would arrive 600 years from now (the 500-year travel time plus the 100 years we had to wait for technology to achieve that speed). Therefore, the second and faster spacecraft would arrive 400 years sooner than the 1000-year spacecraft that could leave now.
Imagine being on that first colony ship, the first generations of humans leaving Earth thinking they’ll be humanity’s first interstellar settlers, only to arrive at the destination and find out humans have already been there for 400 years!
In summary, if we wait for technology to improve and then build and send a faster spacecraft, the newer spacecraft could be capable of overtaking the spacecraft that we could send today. In other words, we could wait longer and still arrive sooner. This has been called the incentive trap because it incentivizes us NOT to send an interstellar spacecraft using current technology and instead to wait for technology to improve before embarking on our first interstellar journey.
The incentive trap has even been suggested as an answer to the famous Fermi paradox: no alien civilization has launched colony ships yet because they are always waiting for faster ships! [1]Lenard & Andrews, “Use of Mini-Mag Orion and superconducting coils for near-term interstellar transportation”, Acta Astronautica 61 (2007) 450-458
In a previous series of articles, I described a variety of spacecraft designs that might take us to the nearest star, Proxima Centauri. One of those, called Project Medusa, utilizes existing technology by harnessing the power of our nuclear weapons arsenal to accelerate the spacecraft to 3.3% the speed of light. The Medusa spacecraft could arrive at Proxima Centauri in about 130 years.
Is a 130-year travel time fast enough? Or should we continue to wait until there is a faster option?
In 2017, Rene Heller of the Max Planck Institute for Solar System Research in Germany published an article in Monthly Notices of the Royal Astronomical Society titled “Relativistic generalization of the incentive trap of interstellar travel with application to Breakthrough Starshot.” [2]Heller, Rene, “Relativistic generalization of the incentive trap of interstellar travel with application to Breakthrough Starshot.”
MNRAS Vol 470 pp. 3664-3671 (2017) [pdf] His article presents a mathematical argument that about 22 years is the optimal travel time before beginning our first interstellar voyage.
In this article, I’m going to share Heller’s mathematical argument supporting the conclusion that about 22 years is the optimal travel time. I’ll keep this overview simple, and the finer details of the calculations can be found in three images of my notes accessible at the end of this article.
Exponential Growth of Vehicle Speeds
Heller’s argument revolves around a fascinating observation about the rate at which our vehicle speed records improve.
Below is a plot of historical speed records versus the year in which the record was set. The vehicles plotted include trains, cars, planes, and rockets (color-coded in the plot).
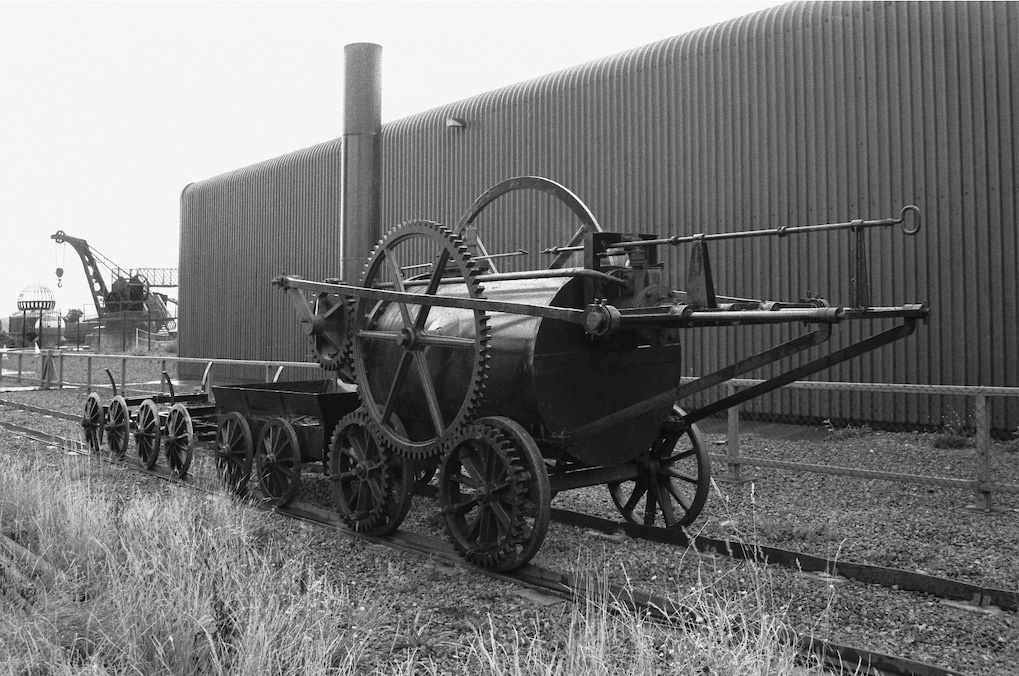
Replica of the Pen-y-Darren, the world’s first steam locomotive, at the Wales National Museum | Image: Amgueddfa Cymru
At the lower left of the plot is the first steam locomotive, the Pen-y-darren from 1804. Its speed was about 4 km/hr (about 2 ½ mph). After Pen-y-darren, rapid gains were made in speed records; trains increased their top speed by more than 10-fold in the 50 years following the Pen-y-darren’s first trip. (Note that the vertical axis of the plot uses a log scale.)
Trains were the only vehicles setting speed records until the invention of cars and planes about 100 years after Pen-y-darren. In 1894, the Benz Velocipede — the world’s first production car — had a top speed of 19 km/hr (about 12 mph).
The Stanley Steamer “Rocket”, 1906
In 1906, the Stanley Steamer steam-powered race car, nicknamed the “Rocket”, reached a record speed of 204 km/hr (127 mph), on par with the fastest trains of the day. Three-feet wide and 16-feet long, it was described as an upside-down canoe made of canvas and glue.
In 1903, three years before the Stanley Steamer’s record, the Wright Brothers’ Flyer debuted with a speed of 11 km/hr (7 mph). Soon after, airplanes overtook the car and train as our fastest form of transportation. About 40 years after the Wright Brothers, airplanes like the German Messerschmitt pushed the world speed record to 1000 km/hr (621 mph).
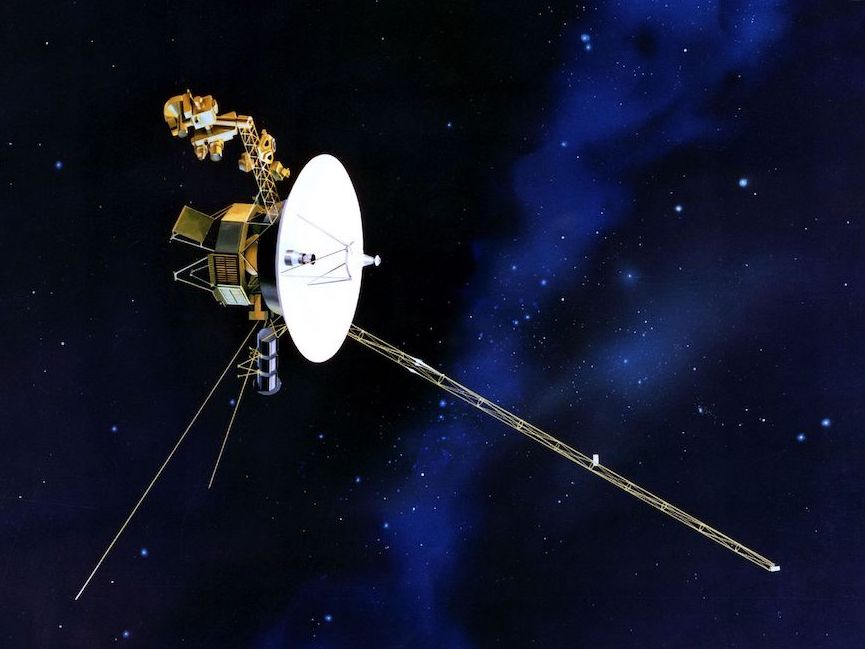
Voyager spacecraft, artist’s rendition | Image: NASA
On the right side of the plot, starting in the 1960’s, rockets become dominant as our fastest vehicles. The Voyager 1 is the first vehicle we’ve constructed that has left our solar system (which happened in 2012) and currently holds the record as the most distant manmade object.
The fastest vehicle we’ve ever built is the Parker Solar Probe. In order to study the Sun’s atmosphere, it has been traveling towards the Sun since 2018 on shrinking orbits, with the Sun’s gravity accelerating it with each smaller orbit. It will eventually (in 2025) reach a speed of over 190 kilometers per second, which is over 400,000 mph. (Even at the speed of 400,000 mph, though, it would take over 7800 years to reach the nearest star, Proxima Centauri.)
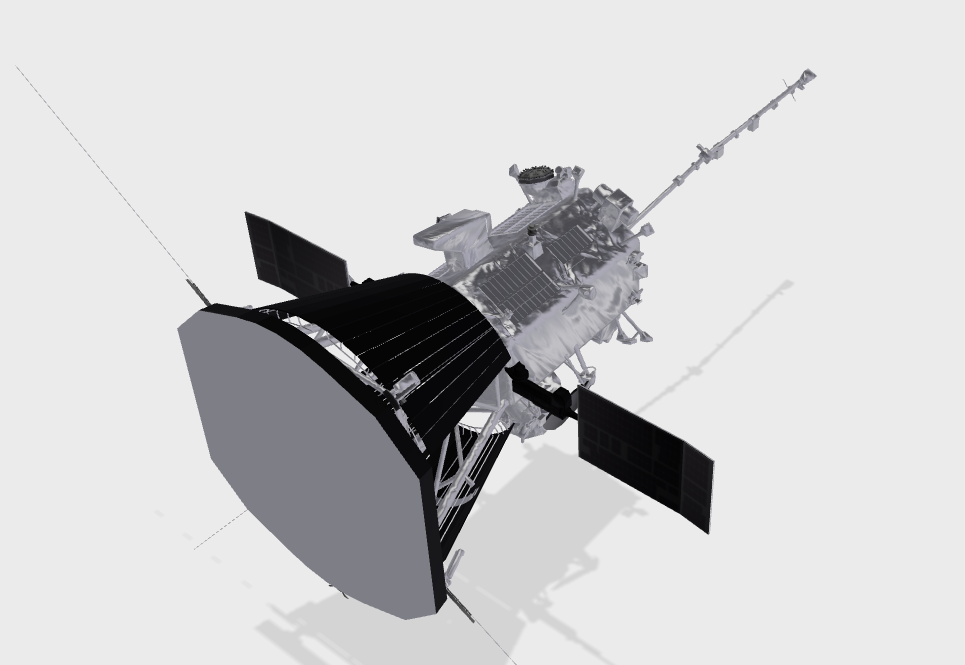
Parker Solar Probe 3D model | Image : NASA
The most remarkable thing about the plot of speed records is the fact that all of these speed records, whether from train, car, plane, or rocket, follow a general trend. A straight line on the plot connects the speed of the Pen-y-darren (in 1804) with the speed of Voyager 1 (as of 2015). The slope of this line is representative of the rate of growth of speed records among all types of vehicles, whether train, car, plane, or rocket.
A straight, increasing line on a semi-log plot like this one is representative of exponential growth. Therefore, our top speed records have been growing exponentially for over 200 years. From this data we can compute that the rate of increase in our top speed has a doubling time of about 15 years. That is: with each new fastest vehicle we invent, it has roughly double the speed of the fastest vehicle from 15 years prior.
A widely-known exponential growth trend is Gordon Moore’s observation — now called “Moore’s Law”, as it has held true since 1975 — that the number of chips on an integrated circuit doubles every two years. The doubling time for transistors on a chip is two years, while the doubling time for vehicle speed records is 15 years. These are both examples of exponential growth.
Anything that is far from the dashed line on the plot is an outlier of the 15-year-doubling rule. You can see on the plot that the Parker Solar Probe is much faster than the 15-year-doubling rule would predict. However, the Parker Solar Probe is not entirely self-propelled, but receives a great deal of assistance from the Sun’s gravity. The Wright Brothers Flyer was slower than the 15-year-doubling rule would suggest, but it was powered by a small 12 horsepower gasoline engine, significantly weaker (though lighter) than the steam engines that dominated speed records at the time.
Calculation of the Optimal Travel Time to Proxima Centauri
With the knowledge that speed records double every 15 years, we can then do the calculation to decide how long we should wait before sending a spacecraft on an interstellar journey.
The total time from the present moment to arriving at the nearest star (call it capital ) is equal to the time we wait (call it lowercase
) plus the travel time. And we know what the travel time will be as a function of how long we wait by using the 15-year-doubling rule.
With this formula in hand, we perform a minimization calculation on using the methods of calculus. Once we’ve found the minimum total time
to the nearest star, we can work backwards to find what the travel time would be at that point in the future.
(You can see the details of the minimization calculation in my notes that are accessible via the thumbnails at the bottom of this article.)
Carrying out the calculation, one finds that the travel time when our minimum total time has been reached will be 21.6 years. Beyond that point, waiting longer would mean arriving later.
In other words: once technology reaches the point where the travel time to Proxima Centauri is 21.6 years, it’s time for us to start our journey there.
Relativity Considerations: The Cosmic Speed Limit
The 15-year-doubling rule can’t go on forever. Eventually, if you keep doubling your speed, you’d reach half the speed of light. Double that again in another 15 years, and you’ve achieved the speed of light. The laws of physics tell us that’s not possible for something with mass. And double it again in another 15 years after that, and you’ve achieved twice the speed of light. Again, that violates the cosmic speed limit.
So modifications have to be made to account for relativity. As you keep increasing your speed, it gets harder and harder to double your speed, in that it takes more and more energy. The energy cost keeps growing, and to achieve the speed of light it would take infinite energy.
Heller did the calculation taking relativity into account as well. It’s a more complicated calculation, and I won’t go through the steps, but the details can be found in my notes at the end of this article.
The important point about the relativistic calculation is that the result is — perhaps surprisingly — approximately the same as the non-relativistic case: once the travel time is about 22 years, it is no longer productive to wait.
When Should We Leave?
To travel to the nearest star (Proxima Centauri) in 21.6 years entails a travel speed of 19.6% the speed of light. How long will it be before we reach that speed of travel? Again, applying the 15-year-doubling rule, we can calculate that it will be about 176 years before we reach that speed.
So it appears that it will take 176 years before it’s time to start our journey to the nearest star — which happens to be remarkably close to the time that elapsed between the Pen-y-darren and the launch of Voyager 1. Combined with the travel time of 21.6 years, that means arriving at Proxima Centauri is at the minimum about 197.6 years in our future.
However, there is a spacecraft design that has been proposed that can reach 20% the speed of light using technology that exists today. The project is called Breakthrough Starshot, and it entails building a spacecraft-on-a-chip that weighs one gram, attaching it to a sail that weighs one gram, and propelling it by shining a powerful laser on the sail. Because its inertia (mass) is so small, and with a laser powerful enough, the spacecraft can be accelerated to 20% the speed of light in a matter of minutes.
I’ve written an in-depth article about Breakthrough Starshot if you’d like more details about that project.
If you look back at the plot of vehicle speed records, you can see Breakthrough Starshot labeled on the graph. It is clearly an extreme outlier, and that’s because the capability of going 20% the speed of light is nearly two centuries ahead of its time.
When will it be time to travel to the nearest star? It appears we’ll have to wait about 176 years for technology to become fast enough. But, if Breakthrough Starshot becomes a reality, the wait will be over and it’ll be time for the human race to go interstellar now.
More Details
The details of Heller’s calculation can be found in my notes, linked here:
Page 1 ![]() |
Page 2 ![]() |
Page 3 ![]() |
Footnotes
↑1 | Lenard & Andrews, “Use of Mini-Mag Orion and superconducting coils for near-term interstellar transportation”, Acta Astronautica 61 (2007) 450-458 |
---|---|
↑2 | Heller, Rene, “Relativistic generalization of the incentive trap of interstellar travel with application to Breakthrough Starshot.” MNRAS Vol 470 pp. 3664-3671 (2017) [pdf] |