Interstellar Ramjets and Antimatter Drives
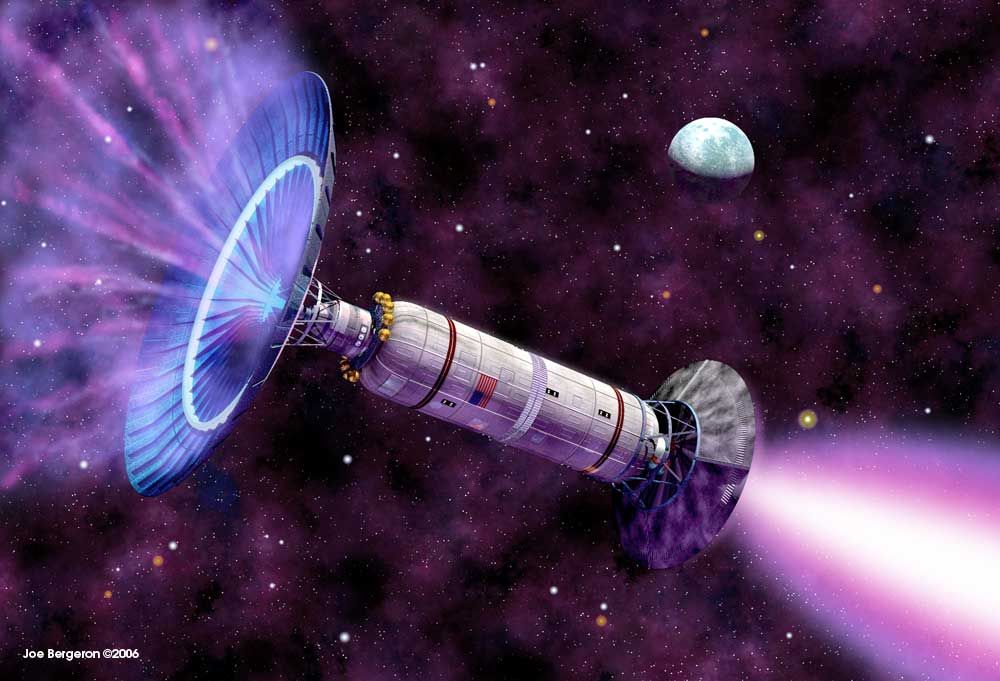
Spacecraft need so much fuel to travel interstellar distances that fuel becomes the only thing they can bring along. Are there any solutions to this problem? This article looks at two: interstellar ramjets and antimatter drives.
Mark Baumann
September 30, 2022
Rockets consume an extremely large amount of fuel. An astronaut once said, “Sitting on top of a rocket is like sitting on top of a Molotov cocktail.” American astronaut Don Pettit tested this statement by calculating that a Molotov cocktail is 52% fuel by mass. For comparison, a Saturn V rocket — which holds the record for heaviest payload carried into Earth orbit — was 85% fuel by mass. Likewise, the Space Shuttle was 85% fuel. Therefore, concluded Pettit, sitting on top of a rocket is far more dangerous than sitting on top of a lit bottle of gasoline.[1]The Tyranny of the Rocket Equation by Don Pettit
To compare with other vehicles: while 85% of a rocket’s mass is fuel, only about 4% of your car’s mass is fuel. A locomotive is 7% fuel, a fighter jet is 30% fuel, and a cargo plane is 40% fuel.
Interestingly, a soda can is 94% soda and 6% can, making it a marvel of modern engineering. However, even that modern marvel is less efficient than the external fuel tank of the Space Shuttle, which was 96% fuel by mass.[2]The Tyranny of the Rocket Equation by Don Pettit
The Spacecraft Fuel Problem
There’s a drawback to carrying lots of fuel. More fuel means more mass. And more mass means it’s harder to accelerate (that’s Newton’s Second Law). Therefore, accelerating requires even more thrust, which requires even more fuel, which further increases the mass, etc. In the words of NASA engineer and award-winning sci-fi author Geoffrey Landis, “If you’re carrying fuel, you need fuel just to carry all the fuel you bring with you.” [3]How to get to Alpha Centauri, NBC News
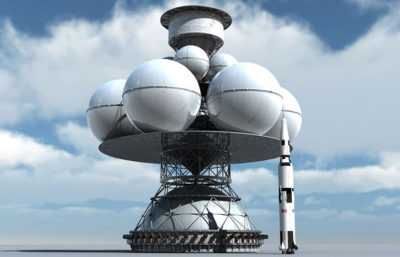
Daedalus spacecraft comparison with Saturn V rocket; the spherical components are fuel tanks | Image: Adrian Mann
Cars, planes, and rockets use chemical reactions to produce energy for propulsion, and chemical reactions won’t get us to the stars (a case that I made in an earlier article through an application of the rocket equation). What if we used nuclear reactions instead? The situation isn’t much better. In that same article, I discussed the Daedalus project, a proposed nuclear-fusion-powered spacecraft that could travel to the nearest star in about 100 years. 93% of the mass of Daedalus is fuel.
There is a formula for determining exactly how much fuel we’d need, given our desired max speed and our exhaust velocity
. The formula is an analog of the rocket equation, but it also accounts for the effects of special relativity. [4]Purcell, Edward. Radioastronomy and Communication Through Space, Brookhaven Lecture Series, num 1, 1960. pdf
Here’s the formula:
where is the mass of the spacecraft plus fuel,
is the mass of the spacecraft without fuel, and
is the speed of light.
Let’s plug in some numbers and see what happens for a nuclear-fusion-powered spacecraft.
Considering a realistic nuclear fusion scenario in which some fraction of the nuclear energy is converted to exhaust energy, we expect the exhaust velocity to be about 3% the speed of light, or nearly 9000 km/s.[5]Zubrin, R. and Andrews, D. Magnetic Sails and Interplanetary Travel, Journal of Spacecraft and Rockets, vol 28, no 2, 1991. pdf (Compare this to the exhaust velocity of a chemical rocket, which tops out at “only” 5 km/s!)
Suppose we want the spacecraft to reach a speed () of 20% the speed of light given it has an exhaust velocity (
) of 3% the speed of light. Then, applying the formula, we get that the fuel to spacecraft ratio is about 58 to 1.
In other words, 98% of the mass of the spacecraft would be fuel at the start of its journey. And that’s just to accelerate to cruising speed and doesn’t include the fuel for slowing down.
The take home point is this: one of the chief obstacles in building an interstellar spacecraft is that we have to take a prohibitive amount of fuel along with us.
Let’s call this the spacecraft fuel problem.
What solutions are there to the spacecraft fuel problem? I can think of three obvious solutions (though there are others):
- Instead of bringing fuel, collect the fuel as you go.
- Get more propulsion from the fuel that you do take, so that you can take less fuel.
- Leave all of the fuel at home as well as the propulsion system itself. Instead, transfer the energy from home to the spacecraft.
In this article I will discuss solutions 1 and 2. Solution 3 will be the topic of the next article of this series of articles on interstellar spacecraft.
Collecting Fuel as You Go: The Bussard Ramjet
How could a spacecraft collect fuel as it goes along? Isn’t space empty? Contrary to popular belief, space isn’t completely empty. The space between stars contains a very dilute gas called the interstellar medium. How dilute? On average the interstellar medium contains about one atom per cubic centimeter. That is extremely dilute. For comparison, a cubic centimeter of air in Earth’s atmosphere has about 30,000,000,000,000,000,000 atoms! So interstellar space is very dilute, but not empty.
What kinds of atoms do we find between stars? Mostly hydrogen.
Hydrogen is the most common element in the universe. The reason why is easy to understand: it’s the simplest element you can make. Being the first element on the periodic table, hydrogen has just a single proton and optionally some additional neutrons and/or electrons. Therefore, even just a lone proton can be thought of as a hydrogen nucleus.
In the simplest type of nuclear fusion, two hydrogen nuclei (each with one proton) collide and “stick” together to make a helium nucleus (with two protons). This process releases large amounts of energy. (For more details about nuclear fusion you can read my first article in this series).
Since hydrogen exists between stars, and hydrogen is also the fuel for the simplest type of nuclear fusion, then why not just collect hydrogen as you travel through interstellar space and use it as fuel in a nuclear fusion-powered spacecraft?
This is the concept behind the Bussard ramjet, proposed in 1960 by American physicist Robert Bussard. [6]Bussard, Robert. Galactic Matter and Interstellar Flight, Astronautica Acta, Vol 6, Fasc 4, 1960 Imagine a spacecraft that has an immense scoop on its nose that can collect hydrogen like a vacuum cleaner as it sweeps through the interstellar medium. It funnels the hydrogen into a nuclear reactor where hydrogen fusion provides power for thrust.

Bussard Ramjet | Drawing by Stan Gibilisco

Bussard Ramjet artist’s conception
The Bussard Ramjet has captured the imagination of both scientists and science fiction authors (artists’ conceptions are depicted here and at the top of this article). It has been mentioned in the works of sci-fi legends such as Poul Anderson (in his famous novel Tau Zero, a story which revolves around a Bussard ramjet that cannot slow down and continues accelerating indefinitely) as well as Hugo Award-winning authors Larry Niven and Vernor Vinge.
Nuclear Power for the Ramjet
Fusing a single proton with another proton is a nuclear reaction that’s called the proton-proton chain (“pp-chain”). Unfortunately, the proton-proton chain isn’t a feasible option for powering a spacecraft because its reaction rate is far too slow, meaning the total number of protons required to get a sufficient outflow of energy is immense. One estimate [7]Whitmire, D. Relativistic Spaceflight and the Catalytic Nuclear Ramjet, Acta Astronautica, Volume 2, 1975. found that the spacecraft would need a nuclear reactor with a radius of 4600 miles (i.e., larger than the Earth!) in order to have enough protons present to produce sufficient thrust. This is why the pp-chain works to power something massive like a star (the Sun is over a million times larger than the Earth by volume) but not something small like a spacecraft.
In 1975, Daniel Whitmire, then at the University of Texas at Austin, revived the feasibility of the ramjet by proposing [8]Whitmire, D. Relativistic Spaceflight and the Catalytic Nuclear Ramjet, Acta Astronautica, Volume 2, 1975. using a different nuclear reaction: the carbon-nitrogen-oxygen (CNO) cycle. In this cycle of nuclear reactions, carbon, nitrogen, and oxygen act as catalysts for the fusion of protons into helium. [9]Here’s the CNO cycle in a nutshell: Start with carbon-12. Carbon-12 fusing with a proton makes nitrogen-13. Nitrogen-13 decays into carbon-13. Carbon-13 fusing with a proton makes nitrogen-14. … Continue reading The catalyst fuel (the carbon, nitrogen, and oxygen) is not depleted and is carried along as part of the ship’s payload. The scooping of hydrogen and the fusion of hydrogen into helium remains the power source for the spacecraft.
The CNO cycle has a much higher reaction rate than the pp-chain (by a factor of ), and therefore it is a feasible power source for an interstellar spacecraft that’s using a nuclear reactor which is much smaller than the core of the Sun.
Accelerating the Ramjet
One nice feature of the ramjet model for propulsion is this: the faster the ramjet goes, the more hydrogen it collides with. More hydrogen means more fuel for its nuclear reactor.
However, how does one get the ramjet started moving in the first place? If the ramjet is at rest, it is not scooping interstellar hydrogen and therefore it has no fuel.
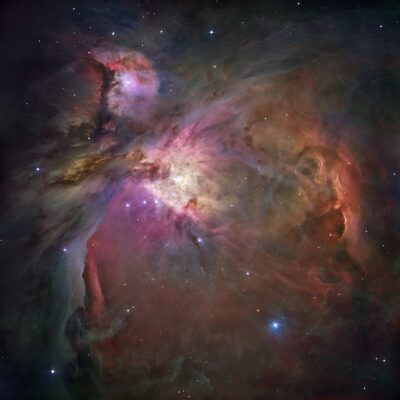
Hubble image of the Orion Nebula, an example region of space where hydrogen may be dense enough to serve as a ramjet runway. | Image: Space Telescope Science Institute
The ramjet could begin its voyage with some hydrogen fuel onboard to get it started. But if we’re required to bring along a lot of hydrogen for the spacecraft to function, then we aren’t solving the spacecraft fuel problem anymore. The question becomes: is the density of nearby interstellar hydrogen sufficient for the ramjet to keep accelerating?
Whitmire [10]Whitmire, D. Relativistic Spaceflight and the Catalytic Nuclear Ramjet, Acta Astronautica, Volume 2, 1975. argues that only a hydrogen nebula is sufficiently dense with hydrogen for the ramjet to accelerate appreciably. He calls such a nebula a “ramjet runway”. Once it reaches sufficient speed using the runway, it can move into less dense regions of the interstellar medium since its increased speed allows it to collect hydrogen at a sufficient rate.
Unfortunately, no such ramjet runway exists in the Sun’s neighborhood. This alone makes the Bussard ramjet impractical for traveling from here to the nearest star, Proxima Centauri. However, this doesn’t rule it out for an alien civilization that happens to live near or in a hydrogen nebula.
Engineering Challenges
Just how big does the ramjet scoop need to be? And how does it funnel hydrogen to the nuclear reactor?
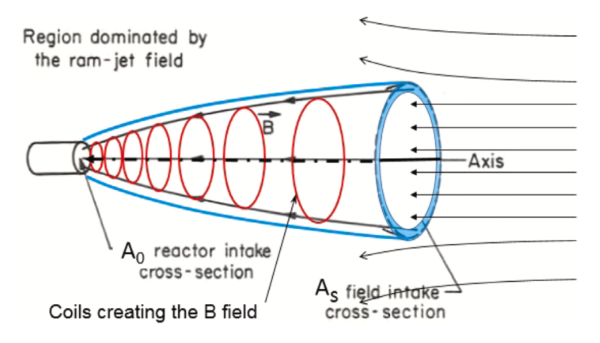
Fishback’s magnetic scoop for the Bussard Ramjet, drawing not to scale | Image from Schattschneider & Jackson (2022) [11]Schattschneider, P. and Jackson, A. The Fishback ramjet revisited, Acta Astronautica 191 (2022) 227-234
In a recent paper from February of 2022, Peter Schattschneider and Al Jackson [13]Schattschneider, P. and Jackson, A. The Fishback ramjet revisited, Acta Astronautica 191 (2022) 227-234 revisit the details of the Fishback magnetic scoop.
Schattschneider and Jackson propose building the magnetic scoop out of a series of superconducting rings that create the magnetic field (these are the red rings depicted in the figure). An electric current flowing around the superconducting rings produces the needed magnetic field.
The faster the ship is moving, the stronger the magnetic field needs to be to corral protons. At extremely high spacecraft velocities, interstellar protons are whizzing by too fast to be captured by a weak magnetic field. Therefore, a faster moving spacecraft requires a stronger magnetic field.
The magnetic field also needs to be parabolic in shape in order to properly funnel protons to the reactor.
Schattschneider and Jackson calculate the necessary size of a scoop that can maintain a magnetic field with the proper shape and strength, and they found that it must be enormous. The scoop (the blue portion in the figure) would have a diameter of 4 km and an overall length of 200 km. That is 600 times longer than the largest aircraft carrier and nearly 5 times taller than the Burj Khalifa, the world’s tallest building. Returning to the diagram above and correcting it for scale, the spacecraft (the black cylinder) should be a mere speck compared to the scoop (the blue portion).
Constructing a vehicle with such dimensions is an engineering challenge far beyond anything we’ve ever attempted.
Matter-Antimatter Annihilation
Let’s turn now to my second proposed solution to the spacecraft fuel problem: could we get more energy out of the fuel we bring along, even more than we get from nuclear fusion? The answer is yes, in principle, using matter-antimatter annihilation.
For every type of matter particle, there is an antimatter particle. There are protons, and there are anti-protons. Electrons and anti-electrons (also called positrons). Quarks and anti-quarks. And so on. Most of the universe is made of regular matter, though antimatter does exist in small quantities. In the rare instance when a matter particle meets one of its antimatter partners, they annihilate each other and release a tremendous amount of energy. How much energy? Let’s compare it to nuclear fusion.
In a fusion reaction, mass is converted into energy, as quantified by the famous equation . In even the most energetic version of a hydrogen-hydrogen fusion reaction, only 0.7% of the mass of the reactants is converted into energy. And yet this is sufficient to power the devastating energy output of a nuclear weapon.
In matter-antimatter annihilation, 100% of the initial mass of the reactants is converted to energy. In other words, the matter and antimatter are annihilated in their entirety and converted completely into energy. This makes matter-antimatter the most efficient form of rocket fuel imaginable.
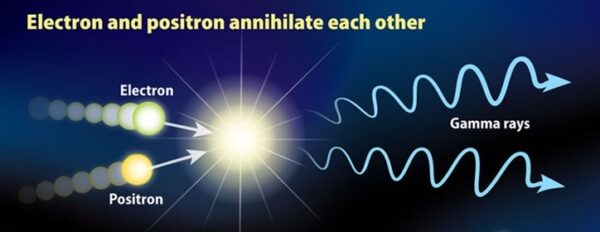
A particle (an electron) meets its antiparticle (a positron) and pure energy emerges (as gamma rays) | Image: Roen Kelly, Astronomy Magazine
To get an idea of the energy that’s available by converting mass to energy at a 100% ratio, consider converting one gram of mass (roughly the mass of a paper clip) into pure energy. How much energy would we get? Apply and the answer is 90 trillion Joules [14]
. That is 1.5x the energy released by the nuclear bomb dropped on Hiroshima, from just a single gram of matter.
This may sound like science fiction, but it’s not. Matter-antimatter collisions happen everyday in our atmosphere. And they are even involved in human technology. Every time someone undergoes a PET (Positron Emission Tomography) scan, for instance, matter-antimatter collisions take place.
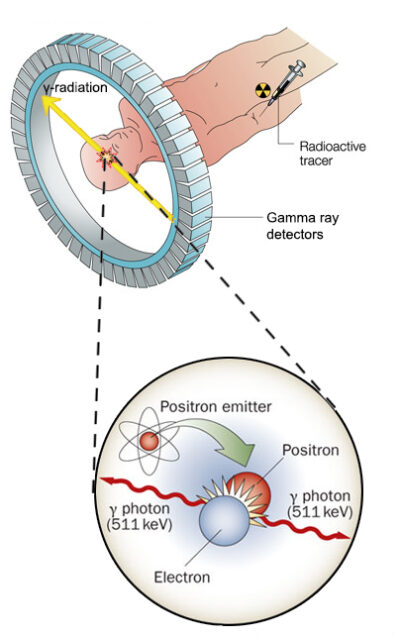
PET scan process | Image: Gabriel Gonzalez-Escamilla
In a PET scan, an unstable nucleus is placed inside the patient. When the unstable nucleus decays, it releases an anti-electron (also called a positron, the P in the PET acronym). The positron travels (on average) about half a millimeter before encountering an electron. They annihilate each other, converting completely into energy, mostly in the form of gamma rays (a high-energy form of light). The gamma rays leave the patient’s body and are captured by a detector. Millions of instances of this — caused by the decay of millions of unstable nuclei — provide enough gamma ray detections to construct a 3D image of the internals of the patient (using a mathematical process called tomography, the T in the PET acronym).
Matter-antimatter annihilation is very much science fact, not science fiction.
Could we power a spacecraft using the conversion of matter to energy via matter-antimatter annihilation?
A spacecraft could carry fuel that is 50% protons and 50% antiprotons. Mixing small quantities of protons and antiprotons would produce tremendous amounts of energy that provide thrust for the spacecraft using a minimal amount of fuel.
Let’s apply the relativistic rocket equation from above to this scenario. Most of the energy produced by matter-antimatter annihilation emerges as light. Therefore, we’ll take the exhaust velocity () to be the speed of light. And as before, we’ll say our maximum desired velocity (
) is 20% the speed of light.
Plugging these numbers into the formula, we find that in order to attain 20% the speed of light, the resulting spacecraft would be 55% fuel at the start of its journey. (Once again, this is for speeding up to cruising speed only, and does not include slowing down.) This ratio is a significant improvement even over chemical rockets that are used to enter low-Earth orbit.
So far, matter-antimatter annihilation seems like a viable energy source for interstellar travel.
However, there are some serious hurdles. I’ll discuss what I think are the two biggest ones: (1) creating antimatter and (2) storing antimatter.
Challenge #1: Creating Antimatter
CERN, in Europe, is the largest particle physics lab in the world. CERN has the capability to produce antimatter. An article on the CERN website explains how they do it: high-energy protons collide with a target and “about four proton-antiproton pairs are produced in every million collisions. The antiprotons are separated from other particles using magnetic fields and are guided to the Antiproton Decelerator, where they are slowed down from 96% to 10% of the speed of light.” [15]Making Antimatter, CERN website

The CERN complex. A few of CERN’s underground particle accelerator experiments are superimposed on the Swiss countryside in their actual locations. The large yellow ring is the Large Hadron Collider (LHC), which has a circumference of 27 km. The LHC particle accelerator ring is the world’s largest ultra-high vacuum chamber. | Image by Maximilien Brice, CERN
According to the same article, the total amount of antimatter produced so far in the history of CERN is about 10 billionths of a gram. Annihilating this much antimatter would provide only enough energy to completely boil away three cups of water. [16]To raise the temperature of a cup (about a quarter liter) of water from room temperature to boiling takes about 84 kJ, and to convert the water to steam requires another 536 kJ, for a total of 620 kJ … Continue reading
If CERN’s accelerators were used for nothing other than producing antimatter, they would produce only about a billionth of a gram per year. In other words, with CERN working around the clock, producing a gram of antimatter would take a billion years. And, as described above, annihilating a gram of antimatter produces roughly the equivalent energy of one-and-a-half nuclear bombs.
Based solely on this information, nuclear weapons appear to be a much more cost-effective solution for storing energy. (Incidentally, there was a proposal in the 1960’s, called Project Orion, for using the explosive power of nuclear weapons to provide thrust for a spacecraft. This was the topic of my previous article, Surfing to the Stars on a Nuclear Blast Wave ).
What would be the monetary cost of producing antimatter? Antimatter production and storage is very inefficient and costly. Again from the CERN website: producing and storing a single gram of antimatter would cost more than a million billion Euros. [17]Making Antimatter, CERN website
A billion years and a million billion Euros for a gram of antimatter. And how much antimatter would a spacecraft need to get to Proxima Centauri? I estimate about 100 kg[18]Using the relationship that annihilating one gram of matter produces the same energy as 1.5 nuclear bombs, combined with the result from my previous article that the Orion spacecraft would need … Continue reading, or 100,000 times as much antimatter as CERN can produce in a billion years.
Clearly, producing enough antimatter to power a spacecraft is beyond the reach of current technology.
Challenge #2: Storing Antimatter
Supposing we could one day make enough antimatter, there is still a storage problem on the spacecraft itself.
Since antimatter will react with regular matter via annihilation, a simple fuel tank made of regular matter won’t suffice. The tank would quickly annihilate with the fuel that it’s meant to carry, and both the tank and its fuel would convert entirely into energy in a tremendous flash of light.
Antimatter can be contained using an electromagnetic field that traps the antimatter in a state of constant spiraling motion so that it never touches the walls of the container. This device is called a Penning trap (for which the Nobel Prize in Physics was awarded in 1989). A Penning trap for antimatter in particular must be built in a vacuum chamber in which no normal matter particles are floating around, since a single proton can annihilate with any antiproton the trap is trying to contain and preserve. Creating a perfect vacuum is notoriously difficult — as you can probably imagine, a single atom can easily sneak in.
At CERN, a Penning trap has been used to isolate and trap antimatter particles. As of 2011, the record length of time for trapping antimatter was 16 minutes. More recently, a trap was able to contain a single antiproton for 57 days before being turned off.

Diagram of Penning Trap used at CERN. The details of how this device works is beyond the scope of this article but you can read more here. | Image from CERN Courier
The 57-day record was for containing a single antiproton. To contain a large amount of antimatter, a larger Penning trap must be built, which means a larger vacuum chamber. How big can we make a vacuum chamber? For the world record we turn, once again, to CERN. [19]A vacuum as empty as interstellar space, CERN website The Large Hadron Collider (LHC) — the world’s largest particle accelerator — is built from ultra-high vacuum chambers, meaning they’re pumped out of air so completely that the gas inside is as rarified as the atmosphere on the Moon. The vacuum chambers total 54 km in length, making the LHC the largest ultra-high vacuum chamber in the world.
Thanks to the Penning trap we can contain antimatter, and in practice have done so (for a single particle) for 57 days. Could we contain large amounts of antimatter, and do so for the many years that an interstellar voyage would require? That remains to be seen.
Furthermore, since the Penning trap is using magnetic fields to trap particles, the apparatus requires magnets. In the words of NASA engineer Geoffrey Landis, “To store antimatter, if you need a ton of magnets for one gram of antimatter, the entire idea of a lightweight way to store immense amounts of energy is no longer lightweight.” [20]How to get to Alpha Centauri, NBC News
While the scientific and engineering challenges of producing and storing large amounts of antimatter are significant, it is at least possible in principle and both producing and storing antimatter have been accomplished at very small scales.
Conclusion
I’ve presented two potential solutions to the spacecraft fuel problem: (1) the Bussard ramjet, which collects hydrogen fuel as it moves through the interstellar medium; and, (2) an antimatter-powered spacecraft that uses matter-antimatter annihilation as its power source.
The Bussard ramjet requires a scoop of immense proportions, and building one is beyond the capabilities of current technology. Furthermore, no hydrogen nebula “ramjet runway” — which has sufficient hydrogen density to provide fuel for the ramjet’s acceleration — exists in the neighborhood of our solar system. As it currently stands, the Bussard ramjet concept won’t be a viable option for getting us to our nearest neighboring star, Proxima Centauri.
An antimatter drive would solve the fuel problem since matter-antimatter annihilation converts mass to energy at the maximal ratio of 100%. Unfortunately, producing enough antimatter is currently far beyond our current technology, and we have yet to build a device that could store large quantities of antimatter for long periods of time. So the antimatter-powered spacecraft is also beyond our current capabilities.
However, not all is lost. I’d mentioned a third potential solution to the spacecraft fuel problem: leave the fuel and the propulsion itself at home, and instead transfer the energy from home to the spacecraft. This will be the topic of the next and final article in this series on interstellar spacecraft.
As you’ll see in the next article, the beamed energy solution is probably the most viable option we have, as it has the potential to get a spacecraft to Proxima Centauri in less than a human lifetime and does so using technology that is currently available to us.
Be sure to check out the next article! And please sign up for my mailing list to be notified when future articles get published.
Footnotes
↑1, ↑2 | The Tyranny of the Rocket Equation by Don Pettit |
---|---|
↑3, ↑20 | How to get to Alpha Centauri, NBC News |
↑4 | Purcell, Edward. Radioastronomy and Communication Through Space, Brookhaven Lecture Series, num 1, 1960. pdf |
↑5 | Zubrin, R. and Andrews, D. Magnetic Sails and Interplanetary Travel, Journal of Spacecraft and Rockets, vol 28, no 2, 1991. pdf |
↑6 | Bussard, Robert. Galactic Matter and Interstellar Flight, Astronautica Acta, Vol 6, Fasc 4, 1960 |
↑7, ↑8, ↑10 | Whitmire, D. Relativistic Spaceflight and the Catalytic Nuclear Ramjet, Acta Astronautica, Volume 2, 1975. |
↑9 | Here’s the CNO cycle in a nutshell: Start with carbon-12. Carbon-12 fusing with a proton makes nitrogen-13. Nitrogen-13 decays into carbon-13. Carbon-13 fusing with a proton makes nitrogen-14. Nitrogen-14 and another proton make oxygen-15, which decays into nitrogen-15. Nitrogen-15 and another proton makes carbon-12 plus a helium nucleus. We are back to where we started (carbon-12) but with an additional helium nucleus present thanks to the input of protons along the way. And then the cycle repeats. |
↑11, ↑13 | Schattschneider, P. and Jackson, A. The Fishback ramjet revisited, Acta Astronautica 191 (2022) 227-234 |
↑12 | Fishback, J.F. Relativistic Interstellar Spaceflight, thesis, MIT, 1969 |
↑14 | ![]() |
↑15, ↑17 | Making Antimatter, CERN website |
↑16 | To raise the temperature of a cup (about a quarter liter) of water from room temperature to boiling takes about 84 kJ, and to convert the water to steam requires another 536 kJ, for a total of 620 kJ to convert a cup of water into steam. 20 nanograms (10 ng each of matter and antimatter) converted directly into energy yields about 1800 kJ, roughly three times the 620 kJ needed to completely vaporize a cup of water. |
↑18 | Using the relationship that annihilating one gram of matter produces the same energy as 1.5 nuclear bombs, combined with the result from my previous article that the Orion spacecraft would need 300,000 nuclear bombs to make the trip to Proxima Centauri, gives the result that we’d need to annihilate 200 kg of matter/antimatter to make the same trip. Splitting this into half matter and half antimatter means 100 kg of each. |
↑19 | A vacuum as empty as interstellar space, CERN website |