A Long Time Ago in Our OWN Galaxy Far, Far Away
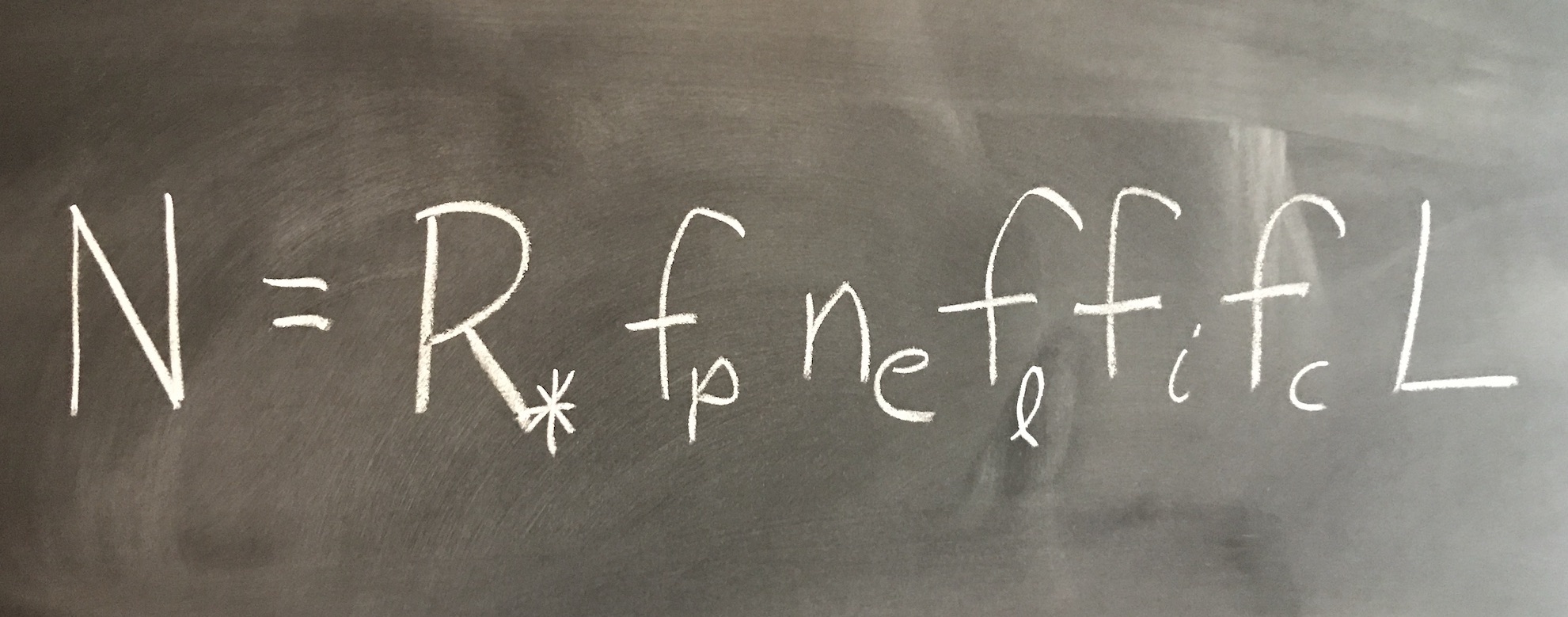
Are we alone in our galaxy? If not, where might other civilizations live in our galaxy? And, when did they live?
Mark Baumann
September 20, 2021
In “A Statistical Estimation of the Occurrence of Extraterrestrial Intelligence in the Milky Way Galaxy”, published in the Jan 2021 issue of the Galaxies journal, the authors draw some interesting conclusions about the existence of other civilizations in our galaxy. (https://arxiv.org/abs/2012.07902)
According to the authors, the proliferation of civilizations in our galaxy likely peaked about 5 billion years ago and was concentrated in a region located about two times closer to the center of the galaxy than where our solar system is located.
If they’re right, then we’re some backwater civilization. And, not only are we far from the action, but we are also so late to the party that most civilizations in the galaxy have already died out.
So let me get this straight: a long time ago, in a very distant place, there was a peak in the abundance of advanced (potentially starfaring) civilizations? George Lucas may have been right all along. Just replace “A long time ago, in a galaxy far, far away” with “A long time ago in our own galaxy far, far away”.
So how did the authors come to their conclusions?
Methodology
The classic way for estimating the number of advanced civilizations in the galaxy is the famous Drake Equation (depicted in the chalkboard image above), which provides a way (with plenty of guesswork) to at least take a stab at estimating a numerical value for the number of advanced civilizations in our galaxy.
This new paper points out right in the introduction that, although useful in some ways, the Drake Equation lacks any time dependence, and therefore cannot model anything that changes over time such as the evolving stellar population in the Milky Way. Certainly the number of stars, metallicity content of solar systems (for forming rocky planets), rate of star birth and death, and frequency of supernova events would influence the evolution of advanced civilizations, and all of those things change over time. Therefore, including a time-dependence in the model makes perfect sense.
This paper takes a Drake-Equation-like approach but augments it with factors that are both temporal (since the galaxy changes over time) and spatial (since not all places in the galaxy are the same). A few values for which we can only take a wild guess are made into adjustable parameters in the model so that the simulation can be run with multiple values in order to see how they vary the outcome. These parameters include:
: the time required for the emergence of life from non-living materials (abiogenesis),
: the time required for the evolution of life to intelligent life, and
: the probability for a civilization to self-annihilate.
Some of these parameters have been investigated in past studies, but the authors claim theirs is the first attempt to incorporate all of these things into one model.
As always with our attempts at understanding the existence and prevalence of extraterrestrial life, we must begin with what we know. And since we’ve only ever seen life on Earth, then a search for life must, at least for now, be a search for life as we know it on Earth.
So, we look for planets that can support life like ours: an Earth-like planet (in size and composition), around a Sun-like star, with an Earth-like orbit (e.g., a mostly circular orbit with non-extreme seasonal variations), in the habitable zone (i.e., at a distance from its star such that water could exist in liquid form). This study makes similar assumptions, and uses the relevant observational information that we have available to us today.
For example, up-to-date observational evidence tells us that 11% of Earth-like planets that we’ve found around other stars are in the habitable zone where liquid water could exist. That, combined with observation-based estimates of the percentage of Sun-like stars and the percentage of those that have Earth-like planets, gives us an estimate of how many habitable (in the sense described above; that is, habitable by Earth-like life) planets might exist in the galaxy at any given time.
Supernova Explosion Rate
Since stars form from gas, the authors can estimate star formation rates using the observed (and curve-fitted) gas density distribution of the galaxy. Where there’s more gas, there’s more material for forming stars. And, thanks to the well-studied life cycle of stars (the main sequence), then given the initial mass of a star we have an excellent estimate of its lifespan. Combining an estimate of the birth rate of stars with their lifespans gives us an estimate on how often stars die. And this leads to an important parameter: the supernova explosion rate.
A supernova explosion occurs when a very large star dies and collapses in on itself. The resulting bounce-back (i.e., the resulting explosion) flings stellar material into space and creates a shock wave that travels through the nearby interstellar medium. A supernova shockwave has the potential to both ignite solar system formation nearby by causing density fluctuations in a region’s gas, as well as bombard existing planetary systems with dangerous radiation and possibly extinguish all life there. For instance, it’s been estimated in a 2003 paper that any planet within about 25 light years of a Type II Supernova will have its land-based life completely exterminated, a process the authors call sterilization.
Model Parameters
, the time it takes for life to become intelligent life, is a poorly understood parameter. On Earth it took about 3.5 billion years. In the study, they use 1, 3, and 5 billion years as values to see how much varying that parameter changes the results.
This parameter is the time it takes for non-living material to become life (abiogenesis). Although this has been reproduced in a lab as long ago as 1952 (Miller-Urey experiment), it is not well understand just how long we should expect this to take in a natural environment. It might be a relatively “quick” process, in fact. On Earth we know from the fossil record that it took a “mere” 300 million years. Compare that to the time it took for simple life to evolve into complex life (3.5 billion years, more than 10 times as long) and it’s plain to see that, at least on Earth, it’s much more likely that simple life will appear than it is that simple life will evolve into complex life (in concordance with the Rare Earth Hypothesis).
If a nearby supernova explosion sterilizes life on a planet during either of the time periods above, the evolutionary clock gets reset back to pre-life conditions: life must again arise from non-life (taking time ), and then evolve into intelligent life (taking time
). Resetting the clock once or more on this billion+ year process means it becomes much less likely that such a planet will have been able to evolve intelligent life since the formation of the Milky Way galaxy roughly 13 billion years ago.
So, while supernova explosions are important for triggering star formation and also expelling heavy metals into the intestellar medium where they can later group themselves into rocky planets like Earth, too many supernova explosions would cause too many sterilization events for complex life to form. Some supernova explosions are needed, but not too many.
Finally, this study includes another parameter: the probability of self-annihilation. Little is known about this probability except that, although it could be 0%, we can’t assume that it necessarily will be zero. Some have argued that it is very close to 100%. Therefore, this study allows the parameter to vary between 0 and 99%.
Results
Two of the most significant results of the study are:
- The probability of self-annihilation
has a very significant impact on the number of possible intelligent civilizations in our galaxy, implying the possibility that self-annihilation could be the Great Filter that’s in our future; and
- regardless of the values of the various parameters, most of the results seem to agree on one thing: the timing and location of the peak of civilization in our galaxy was about 5 billion years ago and centered at a radius about halfway from the galactic center as compared to where we are.

In this heat map, you can see where the peaks occur, both in space and time, for the existence of advanced civilizations. Time since the Big Bang (in billions of years) is the vertical axis of each heat map, and distance from the center of the galaxy (in kiloparsecs) is the horizontal axis of each. The white star in each panel indicates Earth’s location in space and time (i.e., now). The parameters being varied here are: (1) from top to bottom, , the length of time required for life to evolve into intelligent life (
= 1, 3, and 5 billion years, from top to bottom), (2) from left to right,
, the probability of self-annihilation (
= 0%, 50%, 99%, from left to right), and (3)
, the rate of abiogenesis (in columns, as labeled at the top of the figure).
You can see from the figure that Earth’s location in time and space (marked by the white star) is always outside of the peak civilization density and, most remarkably, this happens regardless of the values of the adjustable parameters.
More Interesting Results
Even when self-annihilation is removed ( = 0%, the top row of panels in the figure), this results in roughly the same peak time and location as when
is nonzero. In this case, the extinction of species isn’t due to self-annihilation but rather the declining rates of formation of Sun-like stars combined with supernova sterilization effects.
When the probability of self-annihilation is 10% (not pictured in the figure), the maximum age of any civilization is 60 million years, with the majority of them being younger than 10 million years. And as the probability of self-annihilation goes up, the possible current ages of existing civilizations goes down dramatically.
The rate of abiogenesis () had a surprisingly small effect on the outcome. Which implies that, even if it’s extraordinarily unlikely for life to spring forth from nonliving matter, there is still a good chance that intelligent life could evolve on a planet.
The ETI population of the galaxy versus time is a curve that has just one peak. In other words, once the peak has passed, the ETI population of the galaxy only decreases; there are no more “flare ups” of ETI population density. Instead, it’s a steady decline to zero. And, according to their results, we are 5 billion years past the peak and well into the decline of civilizations in our galaxy. It looks like we may have appeared in our galaxy quite long after civilization’s prime.
On the bright side, because the probability of self-annihilation might be high, then although our galaxy’s highest ETI population density is behind us, those civilizations that do exist today might be quite young, and potentially haven’t advanced to the point where they could communicate with us. This would be a potential answer to the Fermi paradox (the famous question posed by Nobel laureate physicist Enrico Fermi: If the galaxy is teeming with advanced civilizations, then why haven’t we seen any of them?).
Concluding Remarks
While this study does propose solutions to the puzzles of the Fermi Paradox and the Great Filter, it does so by concluding we are a backwater civilization that is late to the party.
If we ever do reach the point where we can explore stars (remotely or otherwise) in distant reaches of the galaxy, the implication that there might be many dead alien civilizations out there lends itself to some exciting possibilities for future xeno-archaeologists. Perhaps we could one day find archaeological evidence of an ancient creator race that disappeared billions of years ago (a la the Alien movie franchise), or of billion-year-old battlefields where ancient civilizations once clashed a long time ago in our own galaxy far, far away.